Mediterranean Sea
Literature Review
Overview
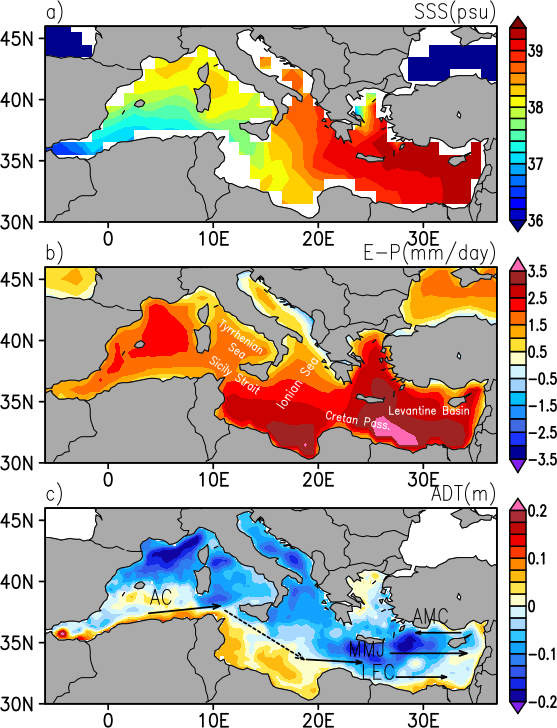
The Mediterranean Sea, a hot spot for climate change (Giorgi, 2006), is characterized by an excess of evaporation over precipitation and river runoff, which is compensated by the entrance of fresher waters from the Atlantic. Significant interest in the Mediterranean Sea is fueled by a hypothesis that this region may be considered as a fast responding laboratory of processes relevant for the global climate change, which regionally develop on relatively short space and time scales (Malanotte-Rizzoli et al., 2014).
Due to strong net evaporation over the Mediterranean Sea, the relatively fresh Atlantic water is modified to become saltier Mediterranean water before eventually returning to the Atlantic through Gibraltar (e.g., Schroeder et al., 2012). Although the positive evaporation-minus-precipitation, largely explains increased Mediterranean salinity levels in comparison to the neighboring salty Atlantic, the spatial pattern of Mediterranean SSS ( see Figure) doesn’t resemble the spatial pattern of E-P suggesting significant impacts of currents and river runoff, and their related horizontal salt transport.
Despite newly available satellite remote sensing salinity for this region, its potential application in the Mediterranean, especially the eastern part, remains widely unexplored. While regional SSS products focusing on the western Mediterranean have been recently released (Isern‐Fontanet et al., 2016; Olmedo et al., 2018), the eastern Mediterranean is still a challenging area for L-band salinity retrieval. High SSS errors are locally present over relatively warm Mediterranean waters. Although the exact nature and magnitude of these regional errors have not been quantified yet, they are attributed to a combination of land contamination (due to multiple islands and complex coastal line) and high level of regional radio frequency interference. These artifacts have stronger impacts on salinity retrievals from SMOS mission, which employs an interferometric L-band radiometer than on those from the SMAP mission.
Despite these artifacts, some new correction/filtering methods were developed (Alvera-Azcarate et al., 2016 ; Olmedo et al., 2018) to provide useful SSS retrievals from SMOS in semi-enclosed basin such as the Mediterranean Sea
Recent studies have indeed demonstrated the potential of new satellite measurements SSS to describe features not well captured by SST maps such as fresh‐core rings in the Mediterranean sea (Isern‐Fontanet et al., 2016).
Analysis of SMOS sea surface salinity data using DINEOF
Given the large amount of missing data (more than 90%) in the eastern part of the North Atlantic Ocean and the Mediterranean Sea, mostly induced by RFI contamination, Alvera-Azcárate et al. (2016) developed a procedure to obtain SMOS SSS data at a daily time step and with a spatial resolution of 0.15° × 0.15° using the DINEOF (Data Interpolating Empirical Orthogonal Functions) method. It has been shown that DINEOF allows retrieving complete daily fields of SSS with reduced noise, by detecting and removing outliers in the initial fields. Constant biases present near land masses, large scale biases and latitudinal biases cannot be corrected with DINEOF because persistent signals are retained in high order EOFs, and therefore these need to be corrected separately.
Improving SMOS Sea Surface Salinity in the Western Mediterranean Sea through Multivariate and Multifractal Analysis
A new methodology using a combination of debiased non-Bayesian retrieval, DINEOF and multifractal fusion has been used by Olmedo et al. (2018) to obtain SMOS SSS fields over the North Atlantic Ocean and the Mediterranean Sea. The debiased non-Bayesian retrieval mitigates the systematic errors produced by the contamination of the land over the sea. In addition, this retrieval improves the coverage by means of multiyear statistical filtering criteria. This methodology allows obtaining SMOS SSS fields in the Mediterranean Sea. However, the resulting SSS suffers from a seasonal (and other time-dependent) bias. This time-dependent bias has been characterized by means of specific Empirical Orthogonal Functions (EOFs). Finally, high resolution Sea Surface Temperature (OSTIA SST) maps have been used for improving the spatial and temporal resolution of the SMOS SSS maps. The presented methodology practically reduces the error of the SMOS SSS in the Mediterranean Sea by half. As a result, the SSS dynamics described by the new SMOS maps in the Algerian Basin and the Balearic Front agrees with the one described by in situ SSS, and the mesoscale structures described by SMOS in the Alboran Sea and in the Gulf of Lion coincide with the ones described by the high resolution remotely-sensed SST images (AVHRR).
Retrieval of eddy dynamics from SMOS sea surface salinity measurements in the Algerian Basin (Mediterranean Sea)
The incoming Atlantic waters into the Mediterranean Sea spread through the basin, affecting its surface circulation [e.g., Millot and Taupier‐Letage, 2005]. In the Algerian Basin, Atlantic waters form a well defined eastward coastal current (Algerian Current, see symbol AC in figure) that can become unstable, generating fresh‐core coastal eddies that propagate downstream. These eddies can detach from the coast but remain trapped in the basin following relatively well defined patterns at distances ∼100–200 km away from the coast. The eddy activity in the region enhances the mixing of the recently entered fresher Atlantic waters with the saltier ambient ones, strongly affecting the spatial distribution of salinity and, therefore, playing a major role in the surface circulation of the Mediterranean Sea. The dynamics of Mediterranean eddies were mainly investigated in the past using Sea Surface Height and SST satellite data [e.g., Taupier‐Letage et al., 2003]. However, Isern‐Fontanet et al. [2014] showed that the direct application of the SQG framework to retrieve currents from SST has some limitations in the Algerian basin due to the seasonal change of the relative orientation of SST and SSS gradients [e.g., Puillat et al., 2002]. Enhancements in the processing of the SMOS data have allowed Isern-Fontanet et al. (2016) to produce, for the first time, satellite SSS maps in the Mediterranean Sea that capture the signature of Algerian eddies. It was shown that SMOS data can be used to track them for long periods of time, especially during winter. SMOS SSS maps are well correlated with in situ measurements although the former has a smaller dynamical range. Despite this limitation, SMOS SSS maps capture the key dynamics of Algerian eddies allowing to retrieve velocities from SSS with the correct sign of vorticity.
Validation of SMAP SSS in the Mediterranean Sea
The SSS retrieved from the SMAP TB at JPL has been validated with in situ measurements from Argo floats, moored buoys, and ship-based TSG data on various time scales in Tang et al. (2017). The unique capability of SMAP to observe salinity signals in coastal oceans and marginal seas was demonstrated through an assessment using TSG data along ship tracks and individual Argo floats in the Mediterranean Sea. The SMAP spatial salinity spatial structure is found to be consistent with the in situ measurements, with better performance in the western Mediterranean than in the east where the effect of RFI (Oriental region) and land contamination (Adriatic Sea) are more severe.